Most of us are probably familiar with pigments. They are, after all, one of the most important components of visual art, and new pigments have been a highly sought-after resource by artists for thousands of years. In both art and nature, they are chemicals that cause skin, hair, eyes, and feathers to reflect certain colors. In humans, melanin is the primary force for skin color. It is also in our hair, eyes, and apparently even our inner ears and brain stem. For us, more melanin is naturally better in some environments, worse in others, and we have evolved as a result. More melanin can help prevent skin cancer, but it can also hinder vitamin D synthesis. Like a great artist, such a simple thing can do so much. In most hair coloration, there are two types of melanin and the ratios of the two types give us most of the amazing array of hair colors we have. A mutation in melanin production for red hair as well as ultraviolet radiation (think of sun bleaching in the summer) add even more variation. Obviously there is much more to this and to human evolution, but this blog post is not about that, it is about art in nature.
In most American birds, feathers are colored either by melanin or carotenoids. For carotenoids, think of carrots, since that is literally what they are named for, and the orange of a carrot is a carotenoid. Typically carotenoids provide the “pretty” colors you see on a bird, while melanin provides the dark feathers. Melanin actually adds strength, so it is more important on flight feathers, especially the tips. If you have ever cracked brown eggs, you probably noticed that they are firmer. This is due to melanin. The dark spots on wild bird eggs? Also melanin.
This need for carotenoids is one reason why nutrition for baby birds is so important, and one reason why even seed-eaters do not feed their young seeds, instead favoring caterpillars and spiders. Also, I’m sure we can all appreciate the concept of having something squishy jammed into our throats over hard seeds, if not the thought of what that squishy thing actually is.
So pigments are great and do a lot of amazing things from giving strength to color to protection. However, they are not the only ways in which wildlife develops color. One very significant and easily overlooked method is structural coloration. Structural colors are colors that come from the physical dimensions of objects that actually cause light waves to interfere with each other, changing the color we see. Everyone is familiar with structural coloration whether or not they realize it. Gasoline is clear; water is clear, but gasoline on water is colorful. This is a result of the structure of the two together. The same goes for soap bubbles you might blow to a child. Specifically this is type of structural coloration—and there are many types—is called “thin-film interference.”
We often think of light reflecting off an object, but don’t consider how, on a clear object, some of the light may reflect off the front of an object while some of the light will travel through the object and reflect off the back side of it. This is actually what happens in the thin film of oil on water, or the thin layer of a soap bubble. The films are very thin, but they still have depth. So some light reflects to your eyes from the front of the film, and some light reflects off the back of the same thin film of soap where it touches water. This is where it gets more complicated.
As we know, light has wavelengths and waves can add or subtract with each other, just like ocean waves on a beach. So light reflecting off the back of the layer can interfere with the light reflecting off the front. As the light hits the film at odd angles–especially as the oil moves–the distance between the back and front changes, so the color you see changes. If the light waves line up perfectly, they will add together to make a stronger reflection at that wavelength. However, if they are exactly opposite, say where one wave is going “up” and the other is going “down,” then they will cancel out and there will be no color at that wavelength. Thus some colors can be stronger while others can be absent, which in turn makes some colors stand out. Of course, rarely will the light be perfectly in-sync like this, especially as the angle of light changes (like as a bubble floats away), but even being close can have a big impact. Thus, we end up with iridescence.
In nature, you have likely unknowingly admired structural coloration in the wings of butterflies and iridescent bird feathers. Wait, you may ask, thin film interference on a butterfly? Where is the oil? Well, many types of structures can create thin film interference. From wing scales on butterflies, to the largely keratin structure of bird feathers, to fish scales and even rocks. I am no expert, but it seems that the more research that is done, the more structural coloration is found. I would be willing to bet that structural coloration in many groups of animals might be the rule rather than the exception. If it isn’t, the numbers are still going to be very impressive.
In order to find the best and simplest examples, I searched for single thin films in nature. Finding them was actually not easy. Most of them are multiple layers, one thin film under another under another, and so on, but we will get to that later. Many insect scales are a single thin film. Micropterix aureatella have fused scales that create a single thin film. Other moths and butterflies have scales that may be multiple thin-films of different types. For instance, many have a lower lamina that is a thin film, separate from whatever structural coloration, pigment, or air gap is happening above it. Many lower laminas of 200 nm give blues to the scale. It seems that thin layers of lamina or wax are about the best I can find of single layers of thin films. It seems insect scales are often stacked however, which kind of removes the whole single thin film from most of the story. Data on species with multiple layers of scales was lacking. Articles just mention scales and not details like how many layers of them there are.

So multilayer thin-film structural coloration is exactly what was described above, it’s more than 1 layer stacked. Light that penetrated through the first layer can still react the same way on the second layer, increasing the structural coloration effects. Wing scales seem to be only a couple to a few deep, so that is not much, but some multilayer thin films can be quite extensive.
Aside from butterflies, one of the the most popular iridescent views in North America is found in hummingbirds. Much of hummingbird coloration is pigment (melanin), but much is also structural thin-film interference. Although birds do produce oil (like we do) to clean their feathers (preen oil), the “thin-film” is created by the structure of their feathers.
For starters, it is important to understand that melanin is produced by organelles in cells called melanosomes. Now, bird feathers are mostly made of keratin, just like our hair and nails. If you think of a feather like a tree, with a main trunk, branches (barbs) coming out of the trunk, and then leaves (barbules) coming off the branches, the barbs and barbules will have melanosomes. In a hummingbird—as well as many other birds like peacocks—they are sometimes structured in a specific way to create iridescence. Specifically, these melanosomes will be relatively flat and wide with air bubbles inside. This is where exciting physics comes into play. Just like the thin-film interference of soap bubbles, melanosomes can create the same thin-film interference due to that bubble. Say light hits a melanosome, and the melanosome reflects some of the red light back out. But some of the light is allowed into the melanosome, through the air bubble, and hit the back end, then reflect red light back out. Because the melanosome is the perfect width for that wavelength, both these reflections of red light end up combining together into a super wave, much like you sometimes see in the ocean. So with the flat and wide melanosomes, if a bird moves slightly and the light reflects at a slightly different angle, then the two waves of red light can match up slightly differently, creating that shimmer as the bird moves.
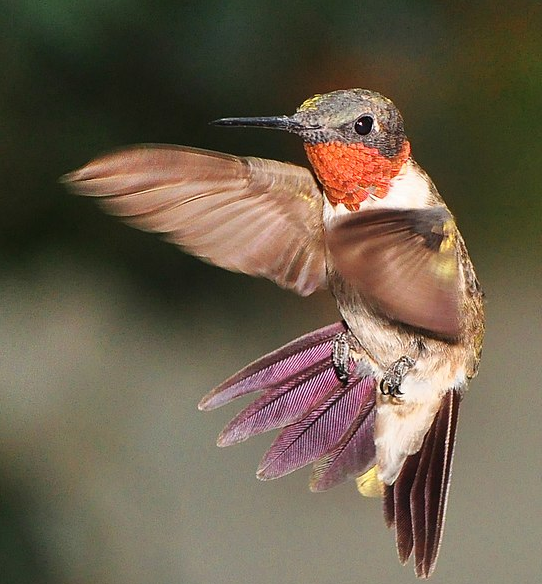
Just as melanosomes can be the exact thickness in order to make a wavelength of light stronger, it can also make waves cancel out. Should a melanosome be half the width of a wavelength, then the reflection off the front and back of the melanosome will be exactly opposite each other and cancel out, essentially preventing that color from being reflected.
Aside from multilayers of feathers or multilayers of melanosomes, structural coloration can also be achieved by the angle of the barbs relative to each other. If the barbs on the right side of a feather are at 180 degrees from the the barbs on the left side, the flash of irridescence can be stronger because they line up the same way. In some birds, the angle is smaller and the shape of the feather is bent backwards, creating a less bright flash. It may be that these birds are still sexually selected for these colors, but overly bright flashes is a signal to predators.
Structural coloration is apparently quite common and there are many types of it, some so technical and microscopic that my two years of physics did not cover enough material to follow. Suffice it to say, it is common. I will make more articles on the subject.
Micropterix aureatella
If the spacing of these layers approaches one-quarter the wavelength of visible light (approx. 380–750 nm), one or more colours will be produced by constructive interference
hummingbird structural coloration is from melanosomes in barbules. If a feather was a tree, then barbules would be the leaves at the end of branches.
The melanosomes’ dimensions and the relative occu-
pation of their components (melanin, keratin and air), together
with the number of melanosome layers, determine the shape
and peak wavelength of the reflectance spectra
Intriguingly, the also species-dependent angle γ formed by
the barbules at either side of the barb stem will additionally
determine the feather reflections. When γ approximates 180°,
as in C. violifer (figure 2h), the beams reflected by the barbules
at both sides of the barb will be virtually parallel, displaying a
single intense flash (figure 2l ). With a more acute angle, as in
C. prunellei, the reflected beams will diverge, permitting two
spatially separated flashes (figure 2k), thus reducing the irides-
cence